Atoms in optical lattices
Fast quantum gas formation via electromagnetically induced transparency cooling
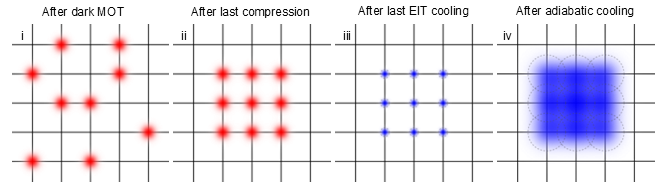

Ultracold quantum gases play a pivotal role in many-body physics, quantum sensing and quantum simulation. Over time, methods such as evaporative cooling in bulk ensembles and precision laser-cooling have been employed to effectively achieve quantum degeneracy in atomic gases. A simpler and more rapid way to form quantum gases would, thus, hold considerable promise in advancing the field. Here, we report the creation of a quantum gas by cooling individual rubidium atoms pinned in a three-dimensional optical lattice using electromagnetically induced transparency and adiabatic expansion. After just 10 ms of cooling, we verified the phase transition from a thermal to a quantum gas by adiabatically transferring the atoms to optical dipole traps. We observed the collapse of atoms in three-dimensional traps, a distinctive hallmark of a quantum gas with negative scattering length. Additionally, in a one-dimensional optical trap, we observed the emergence of a stable and strongly correlated quantum gas. Our results introduce a versatile and fast approach to achieving quantum degenerate gases with minimal time and resource requirements.
Two mode squeezed state in mechanical oscillators
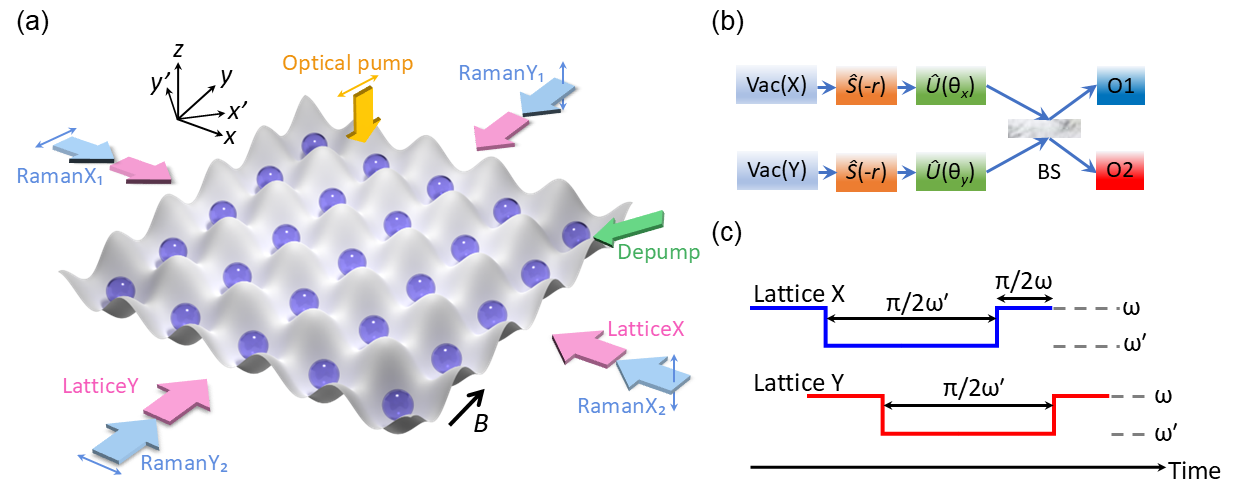
Two-mode squeezed states, which are entangled states with bipartite quantum correlations in continuous variable systems, are crucial in quantum information processing and metrology. Recently, continuous variable quantum computing with the vibrational modes of trapped atoms has emerged with significant progress, featuring a high degree of control in hybridizing with spin qubits. Creating two-mode squeezed states in such aplatformcouldenableapplicationsthatareonlyviablewithphotons.Here,weexperimentally demonstrate two-mode squeezed states by employing atoms in a two-dimensional optical lattice as quantum registers. The states are generated by a controlled projection conditioned on the relative phase of two independent squeezed states. The individual squeezing is created by sudden jumps of the oscillators’ frequencies, allowing generating of the two-mode squeezed states at a rate within a fraction of the oscillation frequency. The states is validated by entanglement steering criteria and Fock state analysis. Our results can be applied in other mechanical oscillators for quantum sensing and continuous-variable quantum information.
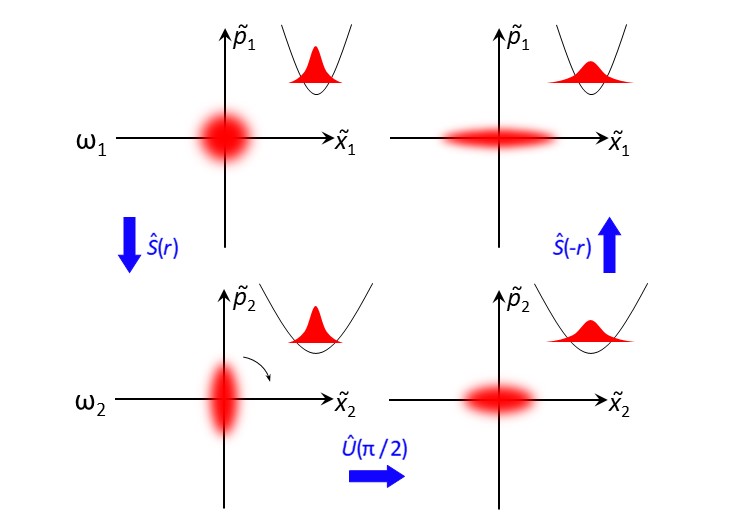
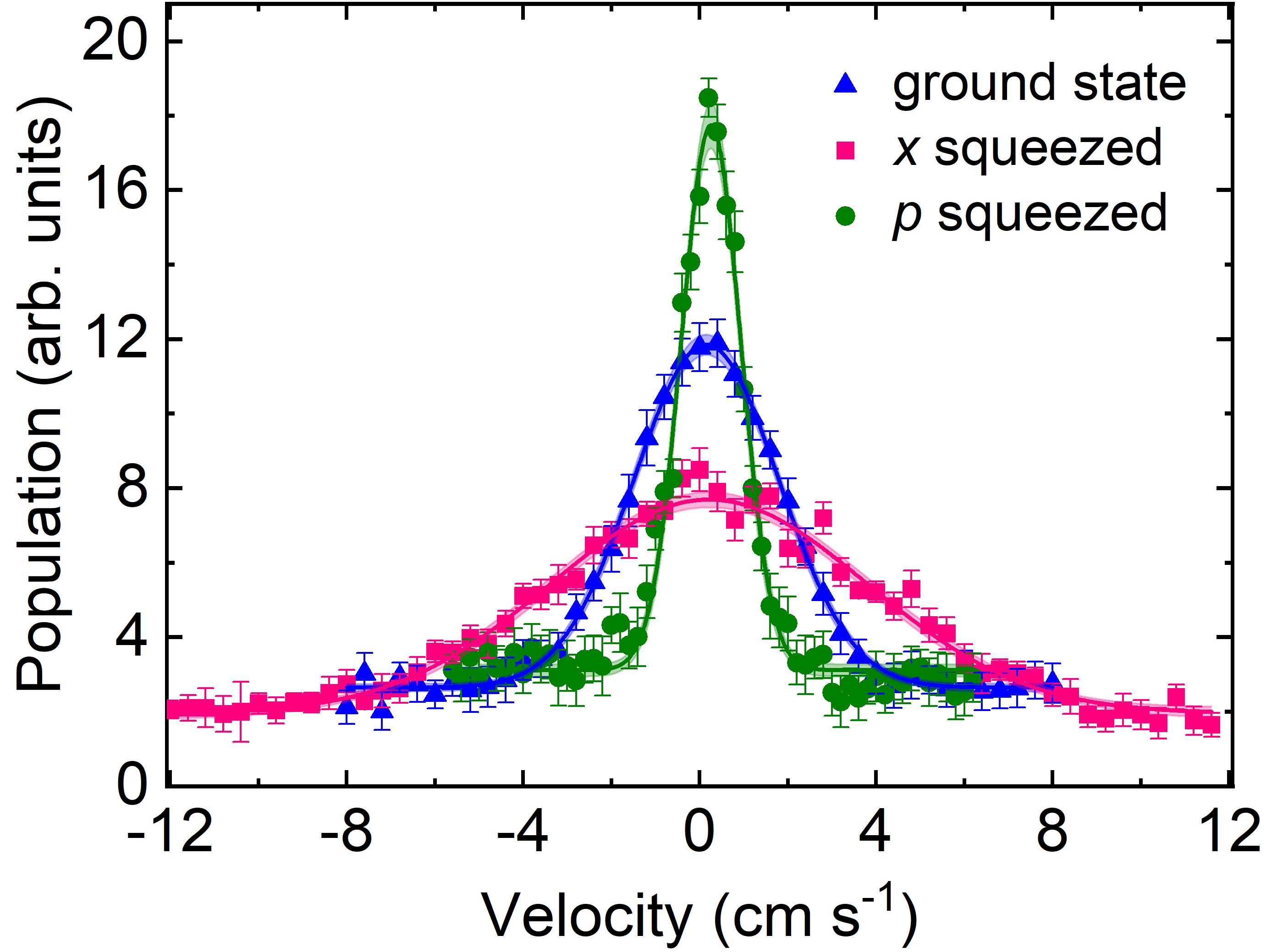
We experimentally demonstrate quantum squeezing in a snap using a harmonic oscillator, a textbook concept that was never realized. Quantum squeezed states lie at the heart of quantum sensing and quantum information processing. However, to create a high fidelity squeezed state, a long creation time in a high quality factor oscillator is necessary to avoid decoherence and errors, limiting the wide usage of quantum squeezed states for practical applications. The frequency jump oscillator has been widely used in discussing the nonadiabaticity of quantum mechanics. The consideration to use this textbook concept for quantum squeezing started in the late 1980s.
The squeezing dynamics in this method do not rely on quantum evolution, and thus the time to create a squeezed state is not limited by the quantum speed limit. Such a fast preparation allows the squeezed state to decouple from decoherence. We verify this in an optical lattice potential where decoherence from anharmonicity prevents the creation of the squeezed state using conventional quantum evolution methods. Our demonstration can be applied to other quantum systems for continuous-variable quantum information to create instantaneous quantum gate operation. We apply this method to amplify a coherent state created by a sudden shift of the potential minimum. The rapid amplification of the coherent state can be used in speeding up quantum dynamics and quantum sensing of motion and force.